R.S. Rigda1,2, L.G. Trahair1,2, T. Wu1,2, T.J. Little1,2, K. Lange1,2, C. Feinle-Bisset1,2, C.K. Rayner1,2,3, M. Horowitz1,2,4, K.L. Jones1,2,4
1. Discipline of Medicine, The University of Adelaide, South Australia, Australia; 2. NHMRC Centre of Research Excellence in Translating Nutritional Science to Good Health, The University of Adelaide, South Australia, Australia; 3; Department of Gastroenterology and Hepatology, Royal Adelaide Hospital, Adelaide, South Australia, Australia; 4. Endocrine and Metabolic Unit, Royal Adelaide Hospital, Adelaide, South Australia, Australia
Corresponding Author: Professor Karen Jones, University of Adelaide, Adelaide Medical School, Adelaide Health and Medical Sciences Building, Cnr North Tce and George St, Adelaide, SA, AUSTRALIA 5005, Tel: + 61 8 83137821, E-mail: karen.jones@adelaide.edu.au
J Aging Res Clin Practice 2018;7:149-155
Published online November 14, 2018, http://dx.doi.org/10.14283/jarcp.2018.25
Abstract
Background: A substantial postprandial reduction in blood pressure (BP), triggered by the interaction of nutrients with the small intestine and associated with increases in heart rate (HR) and splanchnic blood flow, occurs frequently in healthy older people. Objective: The aim of this study was to determine whether these responses are influenced by the length and/or region of small intestine exposed to nutrients. Design: Randomized, single blind study. Setting: Clinical research laboratory. Participants: Ten healthy older participants (9M, 1F; age 65 – 79 yr). Intervention: On 3 separate study days, participants were intubated with a small intestinal catheter incorporating two duodenal infusion ports and an aspiration port, as well as an occluding balloon, which was positioned ~ 60 cm beyond the pylorus. Each participant then received a 60 min (t = 0 – 60 min) intraluminal infusion of glucose (3 kcal/min) into either the proximal (< 60 cm “GP”), or the distal (> 70 cm “GD”), or both (i.e. proximal and distal “GPD”), small intestinal segments. Measurements: BP, HR (automated device), superior mesenteric artery (SMA) blood flow (Doppler ultrasound) and plasma noradrenaline (NA). Results: Small intestinal glucose infusion was associated with reductions in systolic (GP: P = 0.004, GD: P = 0.001, GPD: P = 0.001) and diastolic (GP: P = 0.007, GD: P = 0.004, GPD: P = 0.003) BP and increases in HR (GP: P = 0.001, GD: P = 0.001, GPD: P = 0.002) and plasma NA (GP: P = 0.001, GD: P = 0.002, GPD: P = 0.001), without any difference between the three days. Conclusion: In healthy older participants, the effects of small intestinal glucose to decrease BP and increase SMA flow in healthy older participants appear to be independent of the region, or length, of small intestine exposed.
Key words: Postprandial hypotension, splanchnic blood flow, small intestinal infusion, sympathetic activity.
Introduction
Postprandial hypotension, usually defined as a fall in systolic BP of ≥ 20 mmHg within 2 hours of a meal, is an important clinical problem (1-3), representing a major cause of morbidity, as well as mortality (4). The mechanisms underlying postprandial hypotension are heterogeneous and poorly defined, and current management is unsatisfactory. Postprandial hypotension occurs frequently in apparently healthy older people, as well as nursing home residents, and is associated with diseases characterised by impaired autonomic function, including type 2 diabetes (T2DM) and Parkinson’s disease (3-6). Postprandial increments of noradrenaline (NA), for example, are attenuated in postprandial hypotension (7, 8). In healthy young people, meal ingestion does not affect blood pressure (BP) and, in the broadest sense, postprandial hypotension can be considered to reflect inadequate cardiovascular compensation, including an inadequate sympathetic response, for the postprandial increase in splanchnic blood flow (3, 9).
Studies by ourselves and others have established that gastric distension (10-13), and the subsequent delivery of nutrients to the small intestine (5, 9, 14-17), are major determinants of the postprandial fall in BP. In both healthy older participants (15) and patients with T2DM (5), the hypotensive response to glucose is greater when gastric emptying is relatively more rapid. We have employed direct infusion of nutrients into the duodenum, within the normal range of gastric emptying (1 – 4 kcal/min), to ‘bypass’ the potential effect(s) of gastric distension, and demonstrate that carbohydrate, fat and protein all elicit a fall in BP (18). We have also demonstrated that the relationship between nutrient delivery and the magnitude of the fall in BP is non-linear in healthy older participants (19). The latter may reflect differences in the length of small intestine exposed to glucose; glucose infused at 1 kcal/min is primarily absorbed in the proximal small intestine, while higher rates exceed the glucose absorptive capacity of the duodenum and result in exposure of increasing lengths of small intestine. That the hypotensive response to glucose is secondary to the interaction of nutrients with the small intestine is supported by the observation that when glucose is infused directly into the small intestine in the presence of guar, which is known to slow small intestinal absorption, the magnitude of the fall in BP in healthy older participants is attenuated (20). The outcomes of these studies, accordingly, support the concept that dietary and/or pharmacological strategies that slow gastric emptying and/or small intestinal absorption may be effective in the management of postprandial hypotension (16, 17, 21).
Studies conducted by Lin and colleagues, as well as others, have established that the rate of gastric emptying is dependent on both the length and region of small intestine exposed to nutrients (22-24). Certainly, gastric emptying is regulated primarily by inhibitory feedback arising from the interaction of nutrients with the small intestine, rather than ‘intragastric’ mechanisms. This is likely to reflect, at least in part, major differences in the secretion of hormones between the regions of the intestine, e.g. cholecystokinin (CCK) (25) and glucose-dependent insulinotropic polypeptide (GIP) (26) are released predominantly from the proximal small intestine, while glucagon-like peptide-1 (GLP-1) (26) and peptide YY (PYY) (27) are secreted predominantly in the terminal ileum and colon. We have reported that the release of GLP-1, but not CCK or GIP, by enteral glucose is dependent on the length of small intestine exposed to glucose (25). While this study was unable to distinguish between the effects of length and region of small intestinal exposure, we recently reported in healthy older participants that GLP-1 secretion is primarily dependent on exposure of the distal small intestine to glucose, whereas both proximal (~12 cm post-pylorus) and distal (~70 cm post-pylorus) small intestinal exposure to glucose stimulate GIP secretion comparably (28). There is evidence that a number of gut hormones, including GLP-1 (21, 29), glucagon-like peptide-2 (GLP-2) (30), GIP (31), calcitonin gene-related peptide (CGRP) (32) and neurotensin (33), modulate splanchnic blood flow and may, accordingly, be important determinants of the hypotensive response to a meal. There is no information as to whether the effects of small intestinal nutrients on BP and superior mesenteric artery (SMA) blood flow are dependent on the region and/or length of small intestine exposed, which has implications for the pathogenesis and, hence, rational management of postprandial hypotension.
Using data collected in our previously reported study evaluating incretin hormone release (28), we now report the effects of exposing different regions of the small intestinal to glucose on BP, SMA blood flow and NA in healthy older participants.
Materials and methods
Participants
Thirteen healthy older males and one female [median age 71.3 yr (range: 65-79 yr), body mass index 26.1 kg/m2 (range 22.3–30.1 kg/m2)] were recruited by advertisement. All participants were non-smokers and consumed < 20 g alcohol/day. None had a history of epilepsy, gastrointestinal disease or surgery, diabetes, significant respiratory, renal, hepatic or cardiac disease, or was taking medication known to influence blood pressure or gastrointestinal function.
Protocol
The protocol was approved by the Human Research Ethics Committee of the Royal Adelaide Hospital, and each participant provided written, informed consent. All studies were carried out in accordance with the Declaration of Helsinki.
Each participant was studied on three occasions, separated by at least 7 days in randomised order. On each study day, the participant attended the laboratory at 0800h following an overnight fast (14h for solids, 12h for liquids) (25, 28). A multi-lumen silicone catheter (4.2 mm outer diameter; Dentsleeve International Ltd, Mui Scientific, Mississauga, Canada) was introduced via an anaesthetised nostril (25, 28). The assembly included two infusion channels, an aspiration channel, two ‘air return’ channels, and a balloon (4 cm long, maximum volume ~ 60 mL). The catheter was positioned so that infusion channel 1 opened in the duodenum ~ 12 cm distal to the pylorus, and infusion channel 2 opened ~ 70 cm distal to the pylorus. The balloon was located at ~ 60 cm distal to the pylorus, and was designed to occlude the lumen and thereby ‘isolate’ proximal and distal segments (25, 28). The two aspiration channels opened ~ 3 cm above the balloon, and were used to aspirate infused fluid. The two ‘air return’ channels each opened at three side holes spaced at 5 cm intervals between the balloon and the opening of infusion channel 1, and prevented the duodenal mucosa obstructing the opening of the aspiration channel (25, 28). The correct positioning of the catheter was maintained by continuous measurement of the transmucosal potential difference (TMPD) at saline-perfused sideholes opening in the antrum (- 40 mV) and duodenum (0 mV), using a saline-filled subcutaneous cannula in the left forearm as a reference electrode (25, 28, 34). Intraduodenal infusions were performed using a volumetric infusion pump (Gemini PC-1, IMED, San Diego, CA, USA). An intravenous cannula was positioned in a left antecubital vein for blood sampling, and an automated BP cuff was placed around the right arm. Each participant remained in a supine position while blood sampling, and measurements of BP, HR and SMA blood flow, were performed.
After correct positioning, the balloon was inflated slowly with air using a hand-held syringe until participants reported a sensation of pressure, without discomfort (volume ~ 35 mL) (25, 28). The balloon was then deflated and the participants allowed to rest for ~15 min. The balloon was then re-inflated slowly to the pre-determined volume. The time at which the balloon was fully inflated to the desired volume was defined as t = 0 min. Throughout the study, pressure in the balloon was monitored continuously (25, 28).
Between t = 0 – 60 min each participant received one of the following 3 infusions, in random order, via infusion channels 1 and 2:
a) infusion channel 1: 25% glucose at 3 kcal/min + infusion channel 2: 0.9% saline (ie. proximal small intestinal segment only exposed to glucose; “GP”),
b) infusion channel 1: 25% glucose at 3 kcal/min + infusion channel 2: 25% glucose infused at an adjustable rate to allow the glucose recovered from the proximal segment to be infused concurrently (ie. both the proximal and distal segments exposed to glucose; “GPD”), or
c) infusion channel 1: 0.9% saline + infusion channel 2: 25% glucose at 3 kcal/min (ie. distal segment only exposed to glucose; “GD”).
On the study day that involved glucose infusions into both proximal and distal segments of small intestine (GPD), the 3 kcal/min glucose infusion was initially infused into the proximal segment only and after 10 min, the luminal glucose concentration of the proximal intestinal segment was then determined from the aspirated fluid and used to calculate the appropriate infusion rate for the distal segment of small intestine (i.e. the amount of glucose that would have reached the distal segment if the balloon had not been present) (28). This rate was re-calculated and adjusted every 10 minutes. At t = 60 min, the infusions ceased, the subcutaneous reference was removed, the balloon deflated and the duodenal assembly removed (25, 28). Each participant was then offered a lunch before they were allowed to leave the laboratory. On one day, cardiovascular autonomic nerve function was evaluated immediately after the completion of the study (35, 36).
Measurements
Blood pressure and heart rate
BP (systolic and diastolic) and HR were measured using an automated oscillometric BP monitor (DINAMAP ProCare 100, GE Medical Systems, Milwaukee, WI, USA) immediately before and every 3 minutes after balloon inflation between t = 0 – 60 min. “Baseline” blood pressure and heart rate were calculated as the mean of measurements taken at t = – 9, – 6, and – 3 min (6, 9, 10, 21).
Superior mesenteric artery blood flow
SMA blood flow was measured by Duplex ultrasonography (i.e. B-mode and Doppler imaging) using a Logiq™ 9 ultrasonography system (GE Healthcare Technologies, Sydney, Australia) (18, 21). The participant was scanned using a 3.5C broad-spectrum 2.5- to 4-MHz convex transducer before (i.e. t = – 15 min) balloon inflation and the commencement of (i.e. t = – 5 min) intraduodenal infusions, and then at 15-min intervals between t = 0 and 60 min. Blood flow (mL/min) was calculated using the formula: π x r2 x TAMV x 60, where r was the radius of the SMA and TAMV the time-averaged mean velocity (18, 21). The investigator who performed the SMA blood flow measurements was blinded to the study conditions.
Plasma noradrenaline concentrations
Venous blood samples were collected into ice-chilled EDTA-treated tubes. Plasma was separated by centrifugation at 3,200 RPM for 15 min at 4˚C within 10 min of collection and stored at – 80°C until assayed. Plasma noradrenaline (NA) was measured at t = – 15, 30, and 60 min using high-performance liquid chromatography coupled with electrochemical detection (Waters, Milford, MA, USA) (9). The minimum detectable limit was 0.1 nmol/L, and the inter-assay coefficient of variation was 5.2%.
Aspirate glucose concentrations
The glucose concentration in the proximal segment of small intestine was measured every 10 min. At the conclusion of each 10 min epoch, 1 ml of fluid aspirated from this segment was diluted with 49 ml of saline (0.9%) for immediate measurement of the glucose concentration (2300 YSI Stat Plus, Yellow Springs Instruments, Ohio, USA). The glucose concentration in the aspirate was derived by multiplying this figure by 50, which was then multiplied by the volume of aspirate to yield the total amount of glucose in the aspirate. The equivalent glucose load was then infused into the distal small intestine over the subsequent 10 min (28).
Cardiovascular autonomic nerve function
Standardised cardiovascular reflex tests were used to assess autonomic nerve function (35, 36). Parasympathetic function was evaluated by the variation (R – R interval) of HR during deep breathing and the immediate response to standing (“30:15” ratio). Sympathetic function was assessed by the fall in systolic BP in response to standing. Each result was scored according to age-adjusted predefined criteria: 0 = normal, 1 = borderline and 2 = abnormal, for a maximum total score of 6. A score > 3 was considered to indicate autonomic dysfunction (35, 36).
Statistical analysis
Systolic and diastolic BP and HR were analysed as changes in absolute values from baseline. SMA blood flow and plasma NA were analysed as absolute values. Differences between baseline levels on each study visit were analysed using one-way repeated measures ANOVA. The maximum changes in BP, HR, and SMA flow were defined as the greatest change from baseline in each participant at any time point for each treatment and analysed against a change of 0 using a one-sample t-test. The area under the curve (AUC) for t = 0 – 60 min was calculated for systolic and diastolic BP and HR using the trapezoidal rule and analysed by one-way repeated-measures ANOVA.
All analyses were performed using SPSS version 19.0.0 (SPSS Inc., Chicago, IL, USA). Statistical significance was accepted at P value < 0.05, and data are presented as means ± SEM.
Results
The studies were generally well tolerated. One participant vomited approximately 45 min after commencement of the ID infusion on all 3 study days and was excluded from all analyses. Two participants withdrew because of discomfort during the study. None of the remaining 10 participants (9M,1F; age 70.3 (range 65 – 79) yr; BMI 25.7 (range 22.3 – 30.1) kg/m2) had definite autonomic neuropathy (mean score 0.4, range: 0 – 1). There was no difference in the cumulative (t = 0 – 60 min) glucose recovery between GP (78.1 ± 14.5 kcal) and GPD (70.0 ± 15.9 kcal, P = 0.42). However, because of the aspiration process, the proximal small intestine was only exposed to a mean of 101.9 ± 2.56 kcal on the GP day, rather than the 180 kcal received on the GD and GPD days. Plasma NA samples of 3 participants were compromised because of a freezer malfunction, so that data for plasma NA were analysed for only 7 participants.
Systolic and diastolic BP and HR
There was no significant difference in BP or HR before (baseline) or after (t = 0 min) the balloon was inflated between the 3 days (Table 1).
There was a fall in systolic BP during each infusion (maximum fall in systolic BP from baseline for GP: -11.5 ± 3.0 mmHg; P = 0.004, GD: -14.0 ± 2.1 mmHg; P = 0.001, GPD: -12.2 ± 2.2 mmHg; P = 0.001), with no difference between the study days. There was also a fall in diastolic BP during each infusion (maximum fall in diastolic BP from baseline for GP: -6.3 ± 1.8 mmHg; P = 0.007, GD: -7.2 ± 1.9 mmHg; P = 0.004, GPD: -7.8 ± 2.0 mmHg; P = 0.003), again with no difference between the study days. Similarly, between t = 0 – 60 min, there was no difference in the AUC of the change in either systolic or diastolic BP from baseline between the three study days (Fig 1A + B) or the absolute values in systolic or diastolic BP at 60 min (Table 1).
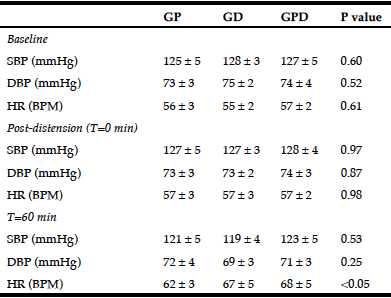
Table 1
Systolic and diastolic blood pressure and heart rate at baseline, in response to balloon distension and at 60 minutes after the intraduodenal infusion
GP, proximal glucose infusion; GD, distal glucose infusion; GPD, proximal and distal glucose infusion respectively; SBP and DBP, systolic and diastolic blood pressure; HR, heart rate. Data presented as mean values ± SEM.
There was a rise in HR during each infusion (maximum rise in HR from baseline for GP: 9.5 ± 1.1 BPM; P = 0.001, GD: 12.5 ± 2.3 BPM; P = 0.001, GPD: 13.5 ± 3.0 BPM; P = 0.002), such that the rise was progressive on the GD and GPD days, but plateaued after ~ 30 min on the GP day. At t = 60 min, HR was lower for GP when compared to GPD (P < 0.05), with a trend to be lower than GD (P = 0.12) and with no difference (P = 1.0) between GD and GPD (Table 1). However, the AUC for HR did not differ significantly between the three study days (Fig 1C).
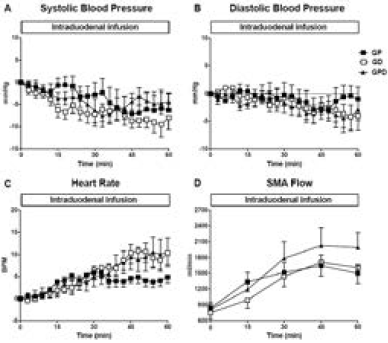
Figure 1
Changes from baseline in (A) systolic blood pressure, (B) diastolic blood pressure and (C) heart rate and (D) absolute superior mesenteric artery (SMA) blood flow in response to intraduodenal glucose infusions into either the proximal, distal or both proximal and distal small intestine. Data are mean values ± SEM
SMA blood flow
There was no significant difference in SMA blood flow before (baseline, Table 2) or after (t = 0 min) the balloon was inflated between the 3 days (Fig 1D).
In response to glucose infusions, SMA blood flow increased on all study days (maximum rise in SMA flow from baseline for GP: 1702 ± 193 ml/min; P = 0.001, GD: 1823 ± 259 ml/min; P = 0.001, GPD: 2120 ± 324 ml/min; P = 0.001) and tended to plateau after ~ 30 min, with no difference between study days (Fig 1D).
Plasma NA concentrations
There was no significant difference in plasma NA before (baseline, Table 2) or after (t = 0 min) the balloon was inflated between the three days.
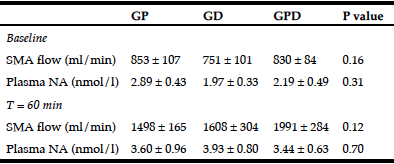
Table 2
SMA blood flow and plasma NA concentrations at baseline and at 60 min after commencement of intraduodenal glucose infusion
GP, proximal glucose infusion; GD, distal glucose infusion; GPD, proximal and distal glucose infusion respectively; SMA, superior mesenteric artery; NA, noradrenaline. Data presented as mean values ± SEM.
There was a progressive rise in NA during each infusion from baseline (Fig 2) with no difference between study days.
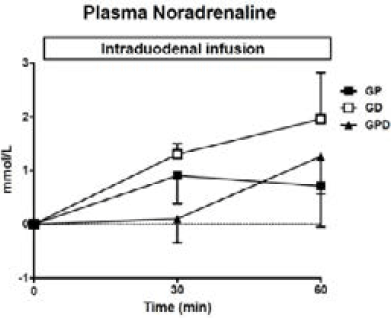
Figure 2
Plasma noradrenaline concentrations during intraduodenal glucose infusions into either the proximal, distal or both proximal and distal small intestine. Data are mean values ± SEM
Discussion
Our study design allowed discrimination between the effects of length and region of small intestine exposed to glucose on BP, SMA flow and plasma NA responses, in healthy older participants. The rate of glucose infusion (3 kcal/min) was at the upper end of the normal range of gastric emptying (37) and known to induce a substantial fall in BP and increases in HR, SMA flow and plasma NA in healthy older participants (9). While we observed that the three permutations in small intestinal glucose delivery were all associated with these effects, supporting the concept that nutrient-gut interaction is an important trigger of postprandial cardiovascular responses, there was no difference in the magnitude of the responses. It is possible that a maximum response was reached with the 3 kcal/min infusion and that differences between length and region of small intestine exposed may be observed with lower infusion rates, however, this appears unlikely. Accordingly, neither the length, nor region of small intestine exposed to nutrient appears to be a substantial determinant of the postprandial hypotensive response.
Our study was stimulated by the recognition that the small intestinal regulation of gastric emptying of nutrients exhibits both length- and region-specificity, clearly shown in the animal studies of Lin and Meyer (22, 23). The concept that the regional distribution of nutrients in the small intestine may influence the BP response was also strengthened by our observations that the α-glucosidase inhibitor, acarbose, diminishes the hypotensive response to oral (38) and intraduodenal (39) sucrose, and may be effective in the management of postprandial hypotension. Furthermore, after Roux-en-Y gastric bypass surgery for morbid obesity, the rate of entry of nutrients, particularly liquids, into the small intestine is usually much more rapid, leading to marked GLP-1 secretion (40), and frequently associated with hypotension (40, 41). Our previous report demonstrated marked region-dependent differences in the stimulation of gut hormone secretion; exposure of glucose to the distal small intestine was associated with substantial GLP-1 secretion, but minimal GIP and CCK responses, while proximal exposure resulted in minimal GLP-1 response, but substantial increases in both GIP and CCK (28).
While we only studied a relatively small number of healthy older participants, our observations were consistent – specifically, there was no suggestion of a differential response in relation to either the fall in BP or rise in plasma NA, the latter being a probable determinant of the postprandial hypotensive response. We recognise that the plasma NA levels are an indirect measure of sympathetic response, reflecting concentrations in the forearm muscle, but are generally recognised as useful (42). While we did not observe a significant difference in SMA flow, given the small subject numbers, it is possible that the trend (P=0.12) for a difference in SMA flow observed between the GPD study day compared to both the GP and GD days represents a type 2 error and this warrants further investigation. The plateau in HR on the GP day from approximately 30 minutes, resulting in a lower HR compared to the GPD day, is not unexpected and may reflect the differences in the amount of glucose infused due to aspiration. Glucose was infused for only 60 min, but a substantial fall in BP, as well as rises in HR and SMA flow, were evident during this time, comparable to observations with similar rates of small intestinal glucose infusion in previous studies (9, 10, 14). It should also be recognised that the two sites of glucose infusion were only separated by a length of ~60 cm, which may have been insufficient to produce distinctive differences in cardiovascular responses. Further studies, infusing glucose more distally are, therefore, warranted. A placebo/saline experiment was not included for logistical reasons, but the magnitude of the observed changes was consistent with our previous observations (9, 10, 14). We assume, but do not know, that the responses to different nutrients and mixed meals would be similar – an oral glucose load is a relevant test ‘meal’, as it is frequently used to diagnose postprandial hypotension (3).
In conclusion, the hypotensive response to small intestinal glucose in healthy older participants appears independent of the region or site of small intestine exposed.
Funding: This study was supported by the National Health and Medical Research Council (NHMRC) of Australia. The salary of K.L. Jones was funded by a University of Adelaide Beacon Research Fellowship, the salary of T. Wu by a Royal Adelaide Hospital Research Committee Early Career Fellowship, the salary of C. Feinle-Bisset by an NHMRC Senior Research Fellowship, and the salaries of R.S. Rigda and K. Lange were funded by the NHMRC. Purchase of the Logiq 9 ultrasonography system was supported by an Equipment Grant from the NHMRC, funds from the University of Adelaide, and GE Medical Systems Australia.
Disclosures: TJL has received research funding from Novartis. CR has received research funding from Sanofi, Novartis, Eli Lilly, Merck Sharp & Dohme, and AstraZeneca. MH has participated in the advisory boards and/or symposia for Novo Nordisk, Sanofi, Novartis, Eli Lilly, Merck Sharp & Dohme, Boehringer Ingelheim, and AstraZeneca and has received honoraria for this activity. KLJ has received research funding from Sanofi, Merck Sharp & Dohme, and AstraZeneca. None of the other authors has any personal or financial conflict of interest to declare.
Author contributions: M.H., and K.L.J. were responsible for the conception and design of the research. R.S.R., T.J.L., and L.G.T. performed the experiments; R.S.R., and K.L.J. analysed the data; R.S.R, C.K.R., T.W., C.F-B., M.H., and K.L.J. interpreted the results of the experiment; R.S.R. and K.L.J. drafted the manuscript; all authors edited, revised and approved the final version of the manuscript.
Ethical standard: The protocol was approved by the Human Research Ethics Committee of the Royal Adelaide Hospital, and each participant provided written, informed consent. All studies were carried out in accordance with the Declaration of Helsinki.
References
1. Jansen RWMM, Lipsitz LA. Postprandial hypotension: epidemiology, pathophysiology, and clinical management. Ann Intern Med 1995;122:286-95
2 Mathias CJ. Postprandial hypotension. Pathophysiological mechanisms and clinical implications in different disorders. Hypertension 1991;18:694-704
3 Trahair LG, Horowitz M, Jones KL. Postprandial hypotension: a systematic review. J Am Med Dir Assoc 2014;15:394-409
4 Fisher AA, Davis MW, Srikusalanukul W, Budge MM. Postprandial hypotension predicts all-cause mortality in older, low-level care residents. J Am Geriatr Soc 2005;53:1313-20
5 Jones KL, Tonkin A, Horowitz M, Wishart JM, Carney BI, Guha S, et al. Rate of gastric emptying is a determinant of postprandial hypotension in non-insulin-dependent diabetes mellitus. Clin Sci (Lond) 1998;94:65-70
6 Trahair LG, Kimber TE, Flabouris K, Horowitz M, Jones KL. Gastric emptying, postprandial blood pressure, glycaemia and splanchnic flow in Parkinson’s disease. World J Gastroenterol 2016;22:4860-7
7 Young JB, Rowe JW, Pallotta JA, Sparrow D, Landsberg L. Enhanced plasma norepinephrine response to upright posture and oral glucose administration in elderly human subjects. Metabolism 1980;29:532-9
8 Mathias CJ, da Costa DF, McIntosh CM, Fosbraey P, Bannister R, Wood SM, et al. Differential blood pressure and hormonal effects after glucose and xylose ingestion in chronic autonomic failure. Clin Sci (Lond) 1989;77:85-92
9 Trahair LG, Vanis L, Gentilcore D, Lange K, Rayner CK, Horowitz M, et al. Effects of variations in duodenal glucose load on blood pressure, heart rate, superior mesenteric artery blood flow and plasma noradrenaline in healthy young and older subjects. Clin Sci (Lond) 2012;122:271-9
10 Vanis L, Gentilcore D, Hausken T, Pilichiewicz AN, Lange K, Rayner CK, et al. Effects of gastric distension on blood pressure and superior mesenteric artery blood flow responses to intraduodenal glucose in healthy older subjects. Am J Physiol Regul Integr Comp Physiol 2010;299:R960-7
11 Shannon JR, Diedrich A, Biaggioni I, Tank J, Robertson RM, Robertson D, et al. Water drinking as a treatment for orthostatic syndromes. Am J Med 2002;112:355-60
12 Gentilcore D, Meyer JH, Rayner CK, Horowitz M, Jones KL. Gastric distension attenuates the hypotensive effect of intraduodenal glucose in healthy older subjects. Am J Physiol Regul Integr Comp Physiol 2008;295:R472-7
13 Jordan J, Shannon JR, Black BK, Ali Y, Farley M, Costa F, et al. The pressor response to water drinking in humans : a sympathetic reflex? Circulation 2000;101:504-9
14 O’Donovan D, Feinle C, Tonkin A, Horowitz M, Jones KL. Postprandial hypotension in response to duodenal glucose delivery in healthy older subjects. J Physiol 2002;540:673-9
15 Trahair LG, Horowitz M, Jones KL. Postprandial hypotension is associated with more rapid gastric emptying in healthy older individuals. J Am Med Dir Assoc 2015;16:521-3
16 Jones KL, MacIntosh C, Su YC, Wells F, Chapman IM, Tonkin A, et al. Guar gum reduces postprandial hypotension in older people. J Am Geriatr Soc 2001;49:162-7
17 Russo A, Stevens JE, Wilson T, Wells F, Tonkin A, Horowitz M, et al. Guar attenuates fall in postprandial blood pressure and slows gastric emptying of oral glucose in type 2 diabetes. Dig Dis Sci 2003;48:1221-9
18 Gentilcore D, Hausken T, Meyer JH, Chapman IM, Horowitz M, Jones KL Effects of intraduodenal glucose, fat, and protein on blood pressure, heart rate, and splanchnic blood flow in healthy older subjects. Am J Clin Nut 2008;87:156-61
19 Vanis L, Gentilcore D, Rayner CK, Wishart JM, Horowitz M, Feinle-Bisset C, et al. Effects of small intestinal glucose load on blood pressure, splanchnic blood flow, glycemia, and GLP-1 release in healthy older subjects. Am J Physiol Regul Integr Comp Physiol 2011;300:R1524-31
20 O’Donovan D, Feinle-Bisset C, Chong C, Cameron A, Tonkin A, Wishart J, et al. Intraduodenal guar attenuates the fall in blood pressure induced by glucose in healthy older adults. J Gerontol A Biol Sci Med Sci 2005;60:940-6
21 Trahair LG, Horowitz M, Stevens JE, Feinle-Bisset C, Standfield S, Piscitelli D, et al. Effects of exogenous glucagon-like peptide-1 on blood pressure, heart rate, gastric emptying, mesenteric blood flow and glycaemic responses to oral glucose in older individuals with normal glucose tolerance or type 2 diabetes. Diabetologia 2015;58:1769-78
22 Lin HC, Doty JE, Reedy TJ, Meyer JH. Inhibition of gastric emptying by glucose depends on length of intestine exposed to nutrient. Am J Physiol Regul Integr Comp Physiol 1989;256:G404-G11
23 Lin HC, Doty JE, Reedy TJ, Meyer JH. Inhibition of gastric emptying by sodium oleate depends on the length of intestine exposed to nutrient. Am J Physiol Regul Integr Comp Physiol 1990;259:G1031-G6
24 Fone DR, Horowitz M, Heddle R, Maddox AF, Collins PJ, Read NW, et al. Comparative effects of duodenal and ileal intubation on gastric emptying and postprandial antral, pyloric, and duodenal motility. Scand J Gastroenterol 1991;26:16-22
25 Little TJ, Doran S, Meyer JH, Smout AJ, O’Donovan DG, Wu KL, et al. The release of GLP-1 and ghrelin, but not GIP and CCK, by glucose is dependent upon the length of small intestine exposed. Am J Physiol Endocrinol Metab 2006;291:E647-55
26 Baggio LL, Drucker DJ. Biology of incretins: GLP-1 and GIP. Gastroenterology 2007;132:2131-57
27 Adrian TE, Ferri GL, Bacarese-Hamilton AJ, Fuessl HS, Polak JM, Bloom SR. Human distribution and release of a putative new gut hormone, peptide YY. Gastroenterology 1985;89:1070-7
28 Rigda RS, Trahair LG, Little TJ, Wu T, Standfield S, Feinle-Bisset C, et al. Regional specificity of the gut-incretin response to small intestinal glucose infusion in healthy older subjects. Peptides 2016;86:126-32
29 Bremholm L, Andersen UB, Hornum M, Hilsted L, Veedfald S, Hartmann B, et al. Acute effects of glucagon-like peptide-1, GLP-19-36 amide, and exenatide on mesenteric blood flow, cardiovascular parameters, and biomarkers in healthy volunteers. Physiol Rep 2017;5 :e13102
30 Hansen LB. GLP-2 and mesenteric blood flow. Dan Med J2013;60:B4634
31 Fara JW, Salazar AM. Gastric inhibitory polypeptide increases mesenteric blood flow. Proc Soc Exp Biol Med 1978;158:446-8
32 Mulholland MW, Sarpa MS, Delvalle J, Messina LM. Splanchnic and cerebral vasodilatory effects of calcitonin gene-related peptide I in humans. Ann surg 1991;214:440-5
33 Parker DR, Carlisle K, Cowan FJ, Corrall RJ, Read AE. Postprandial mesenteric blood flow in humans: relationship to endogenous gastrointestinal hormone secretion and energy content of food. Eur J Gastroenterol Hepatol 1995;7:435-40
34 Heddle R, Collins PJ, Dent J, Horowitz M, Read NW, Chatterton B, et al. Motor mechanisms associated with slowing of the gastric emptying of a solid meal by an intraduodenal lipid infusion. J Gastroenterol Hepatol 1989;4:437-47
35 Ewing DJ, Clarke BF. Diagnosis and management of diabetic autonomic neuropathy. Br Med J Clin Res Ed 1982;285:916-8
36 Piha SJ. Cardiovascular autonomic reflex tests: normal responses and age-related reference values. Clin Physiol 1991;11:277-90
37 Brener W, Hendrix TR, McHugh PR. Regulation of the gastric emptying of glucose. Gastroenterology 1983;85:76-82
38 Gentilcore D, Bryant B, Wishart JM, Morris HA, Horowitz M, Jones KL. Acarbose attenuates the hypotensive response to sucrose and slows gastric emptying in the elderly. Am J Med 2005;118:1289.e5-.e11
39 Gentilcore D, Vanis L, Wishart JM, Rayner CK, Horowitz M, Jones KL. The alpha (alpha)-glucosidase inhibitor, acarbose, attenuates the blood pressure and splanchnic blood flow responses to intraduodenal sucrose in older adults. J Gerontol A Biol Sci Med Sci 2011;66:917-24
40 Nguyen NQ, Debreceni TL, Burgstad CM, Wishart JM, Bellon M, Rayner CK, et al. Effects of Posture and Meal Volume on Gastric Emptying, Intestinal Transit, Oral Glucose Tolerance, Blood Pressure and Gastrointestinal Symptoms After Roux-en-Y Gastric Bypass. Obes Surg 2015;25:1392-400
41 Tack J, Arts J, Caenepeel P, De Wulf D, Bisschops R. Pathophysiology, diagnosis and management of postoperative dumping syndrome. Nat Rev Gastroenterol Hepatol 2009;6:583-90
42 Goldstein DS, McCarty R, Polinsky RJ, Kopin IJ. Relationship between plasma norepinephrine and sympathetic neural activity. Hypertension 1983;5:552-9