J.R. Bush1,2, M.J. Alfa3
1. MSPrebiotics Inc., Carberry, MB, Canada; 2. Department of Biology, Brandon University, Brandon, MB; 3. Department of Medical Microbiology, University of Manitoba, Winnipeg, MB, Canada.
Corresponding Author: Jason R. Bush, MSPrebiotics Inc., Carberry, MB, Canada, jbush@MSPrebiotics.com
J Aging Res Clin Practice 2018;7:176-180
Published online December 11, 2018, http://dx.doi.org/10.14283/jarcp.2018.28
Abstract
Objective: To determine whether changes in specific Firmicutes genera are correlated with changes in blood glucose and/or insulin levels in participants consuming MSPrebiotic® digestion resistant starch (DRS). Design: Double-blinded, randomized placebo-controlled trial (September 2013 to May 2015). Participants: Mid-aged (MID) adults aged 30-50 years (42 enrolled, 40 analyzed) and elderly (ELD) adults 70 years or older (42 enrolled, 35 analyzed), including long-term care facility (ELD) and community dwellers (MID and ELD) in Winnipeg, Canada. Intervention: Participants consumed 30 g placebo (digestible starch) daily for 2 weeks and were then randomized to receive 30 g per day placebo or 30 g per day DRS for 3 months. Measurements: Correlations between abundance of all Firmicutes genera detected in the gut microbiome and blood glucose and insulin levels were calculated. Significance was determined using the Benjamini-Hochberg procedure. Results: We report significant correlations between changes in blood glucose and change in Butyricicoccus (r = – 0.59212, p = 0.007578) and change in Sporacetigenium (r = 0.637197; p = 0.003349) in the ELD group consuming DRS, and significant correlations between the changes in blood glucose and change in Roseburia (r = 0.55731, p = 0.013178), change in Sporacetigenium (r = 0.572948, p = 0.010351), and change in Anaerostipes (r = – 0.59026; p = 0.007836) in the MID group consuming DRS. Sporacetigenium abundance at baseline is significantly correlated with insulin levels in the general population (r = 0.337136901; p = 0.003102). Conclusion: Sporacetigenium abundance in the gut microbiome is correlated with diabetic parameters in both MID and ELD groups and may be a microbiome marker that parallels the development of diabetes.
Key words: Sporacetigenium, firmicutes, resistant starch, blood glucose, insulin.
Introduction
While we can now identify gut microbiota to genus or species levels (1), our understanding of their functional activities remains limited. Both Bacteroidetes and Firmicutes phyla are producers of short chain fatty acids, with Bacteroidetes bacteria generally producing acetate and propionate, and Firmicutes bacteria generally producing butyrate (2). Butyrate is both an energy source and a multifunctional signaling molecule, helping mediate dietary influences on various physiological processes (3)(4). Specifically, butyrate stimulates release of glucagon-like peptide-1 (GLP-1), a potent insulinotropic incretin produced by enteroendocrine L-cells in the intestines (5). Previous studies have linked increasing dietary prebiotics to elevated GLP-1 levels and improved diabetic parameters (6), but the role between specific gut microbe genera, including those belonging to phylum Firmicutes, and diabetic parameters remains largely unexplored.
The impact of age-related gut microbiome changes on human health is well-studied (7) and the importance of Bifidobacterium and Lactobacillus abundance in maintaining gut health is known. We previously demonstrated that consumption of prebiotic MSPrebiotic® digestion resistant starch (DRS) stimulated the growth of Bifidobacterium, decreased E. coli/Shigella species, increased butyrate, and improved blood glucose, insulin, and insulin resistance levels in the elderly (8, 9). While Bifidobacterium (phylum Actinobacteria) are potent DRS fermenters, they do not produce butyrate, and we concluded that increased levels of butyrate in feces of ELD consuming DRS were likely due to cross-feeding that stimulated Firmicutes bacteria (3, 8, 10). This may be an “age-related” or “prebiotic-specific” phenomenon, as increasing Bifidobacterium abundance with galactooligosaccharides did not translate to improved insulin resistance in obese prediabetics with a mean age of 59 years (11).
The objective of this study was to determine if specific changes in Firmicutes genera of the gut microbiome correlated with the blood glucose or insulin changes previously identified in ELD consuming DRS.
Methods
Clinical Study, Sample Collection, and Processing
Adults aged 30-50 years (middle-aged cohort; MID; 42 enrolled) or aged 70 years or older (elderly cohort; ELD; 42 enrolled) consumed 30 g per day of placebo (digestible corn starch; Amioca TF, Ingredion, Brampton, ON) for two weeks before being randomized to placebo or MSPrebiotic (digestion resistant potato starch (DRS); MSPrebiotics Inc., Carberry, MB) arms (8, 9). Participants then consumed 30 g of placebo or DRS daily for 12 weeks (14 weeks total). Stool and fasting blood samples were collected at baseline and 14 weeks. Prediabetics and diabetics were excluded based on enrollment screening. Antibiotic treatment alters the microbiome, so only samples from participants who did not receive antibiotic treatment within 5 weeks of stool sample collection were analyzed (MID = 40, ELD = 35). Blood glucose levels were determined by Diagnostic Services Manitoba (Winnipeg, MB) and insulin levels by LipoScience Inc. (Raleigh, NC). Gut microbiome analysis was performed by 16S sequencing on the Illumina MiSeq platform and alignment as previously described (1, 8, 12).
Statistical Analysis
Baseline values were subtracted from week 14 values and expressed as a change in percent (blood glucose and insulin levels) or a change in relative abundance (bacteria) for each participant. Pearson’s correlation coefficients (r) and p values for changes in Firmicutes genera and change in blood glucose in both the MID and ELD cohorts consuming DRS were calculated using Excel (Microsoft, Redmond, WA). Significance was determined using the Benjamini-Hochberg procedure (13) at a false discovery rate (FDR; q) of 0.1. The critical values for each genus were generated by dividing the p value rank (i) by the total number of genera analyzed (m), then multiplying this quotient by the FDR (q). Pearson’s correlation coefficients and p values were calculated using Excel for select changes in Firmicutes genera and changes in insulin levels. Correlations between change in genus abundance and change in insulin level were considered significant at p < 0.05.
Results
We previously observed a significant reduction in blood glucose levels in ELD individuals consuming DRS compared to placebo (9). We sought to determine the relationship between the change in blood glucose and the change in the abundance of Firmicutes genera in the ELD group consuming DRS. Pearson correlation coefficients (r) representing change in blood glucose and change in abundance were calculated for each genus. Positive r values indicate positive correlations (ie. decreasing blood glucose with decreasing bacteria levels) and negative r values indicate negative correlations (ie. decreasing blood glucose with increasing bacteria levels). These data were ranked by significance and, beginning with the lowest ranking genus, each p value was compared to the Benjamini-Hochberg critical value (Table 1).
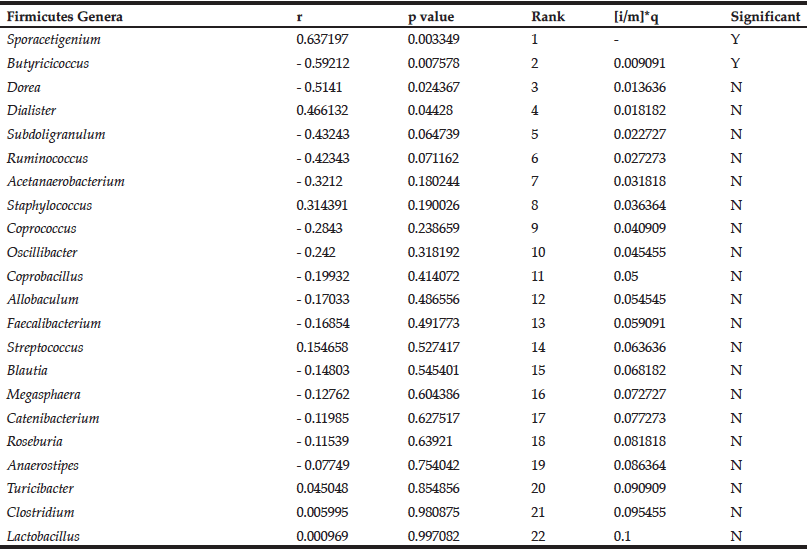
Table 1
Correlations between the change in abundance of Firmicutes genera and change in blood glucose levels in ELD consuming DRS
The Benjamini-Hochberg method was employed to control for false discovery of significant correlations (13). Results are rank ordered based on p value, and the p value is compared to the critical value ([i/m]*q, FDR = 0.1) beginning with the lowest ranking genus (Lactobacillus). The first correlation with a p value lower than the critical value (Butyricicoccus) and all higher-ranking correlations (Sporacetigenium) are considered significant. Positive Pearson correlation coefficient (r) values indicate positive correlations and negative r values indicate negative correlations.
While DRS consumption had no effect on mean blood glucose levels in the MID group (9), some individuals’ blood glucose levels improved over the course of the study. We therefore determined the relationship between blood glucose levels and Firmicutes genera in the MID group consuming DRS. Pearson correlation coefficients representing change in blood glucose and change in abundance were calculated for each genus. These data were ranked by significance and, beginning with the lowest ranking genus, each p value was compared to the Benjamini-Hochberg critical value (Table 2).
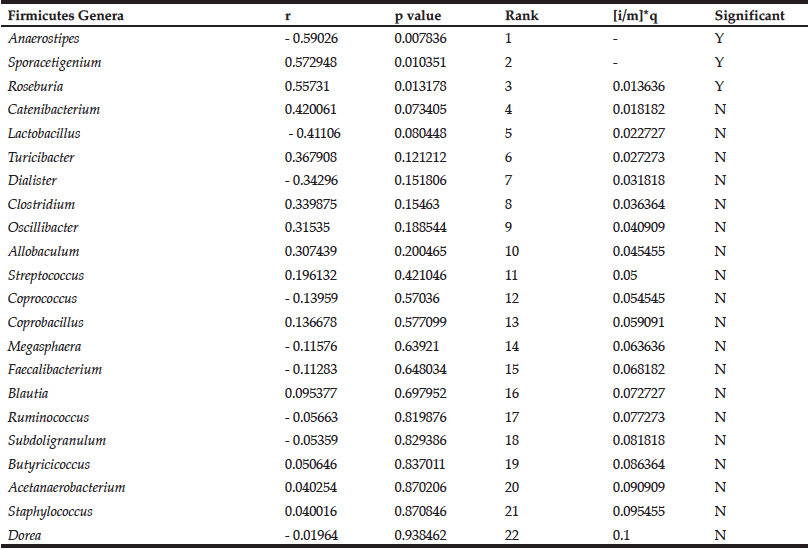
Table 2
Correlations between the change in abundance of Firmicutes genera and change in blood glucose levels in MID consuming DRS
The Benjamini-Hochberg method was employed to control for false discovery of significant correlations (13). Results are rank ordered based on p value, and the p value is compared to the critical value ([i/m]*q, FDR = 0.1) beginning with the lowest ranking genus (Dorea). The first correlation with a p value lower than the critical value (Roseburia) and all higher-ranking correlations (Sporacetigenium; Anaerostipes) are considered significant. Positive Pearson correlation coefficient (r) values indicate positive correlations and negative r values indicate negative correlations.
We also reported lower insulin levels in the ELD group consuming DRS (9). We expected genera displaying a significant correlation with blood glucose to be similarly correlated with insulin levels. We therefore calculated Pearson correlation coefficients representing the change in insulin and change in abundance for the significant genera from Tables 1 and 2. In the ELD group, change in Sporacetigenium and change in insulin levels were significantly correlated (r = 0.668881936, p = 0.001743) and a correlation between change in Butyricicoccus and change in insulin levels trended towards significance (r = – 0.440113219, p = 0.059404). In the MID group, a correlation between change in Sporacetigenium and change in insulin levels trended towards significance (r = 0.435985629, p = 0.080278), but there was no correlation between change in insulin levels and changes in either Anaerostipes (r = – 0.243063987; p = 0.347324) or Roseburia (r = – 0.003057256; p = 0.990883).
Sporacetigenium is normally found in low abundance (Figure 1), so we asked whether high Sporacetigenium levels may be an indicator of elevated blood glucose or insulin in the general population. We calculated Pearson correlation coefficients representing levels of Sporacetigenium and either blood glucose or insulin levels among all participants of the study (ELD and MID, both treatments, N = 75) at baseline. While there was no correlation between Sporacetigenium and blood glucose (r = – 0.051100236; p = 0.663898), levels of Sporacetigenium and insulin were significantly correlated at baseline (r = 0.337136901; p = 0.003102). Given that more insulin is required to maintain normal blood glucose levels in people developing insulin resistance, these data suggest that Sporacetigenium levels in the gut are relevant to overall metabolism. Figure 1
Relative abundance, representing the fraction of all bacteria detected in stool samples, is depicted for genera from the Firmicutes phylum in the MID and ELD groups prior to intervention (mean +/- SD). Genera are ordered from most to least abundant based on MID values.
Discussion
We report correlations between Sporacetigenium (phylum Firmicutes) and baseline insulin levels, and between declining levels of Sporacetigenium and improving levels of blood glucose and insulin in response to DRS consumption. Importantly, correlations between Sporacetigenium and fasting blood glucose levels were detected in both elderly and middle-aged individuals, and no correlations with other genera were detected in both cohorts. We propose that increased Sporacetigenium abundance may be a marker for microbiome-related changes leading to pre-diabetes.
We speculated that reduced blood glucose and insulin levels in DRS consumers could be due to increased butyrate, accompanied by increases in butyrate-producing Firmicutes genera. DRS consumption was previously shown to increase levels of the butyrate-sensitive hormone GLP-1 (6). Consistent with this hypothesis, we detected a significant correlation between changes in blood glucose and changes in the butyrate producer Butyricicoccus and a near-significant correlation between insulin and Butyricicoccus in the elderly cohort. Our previous study demonstrated that DRS consumption led to increased butyrate levels in the ELD but not MID group (8), so the lack of correlation between Butyricicoccus and either blood glucose or insulin in the middle-aged cohort is unsurprising.
Sporacetigenium was originally identified from strains isolated from an anaerobic digester treating municipal waste and sewage in Fujian, China (14). While very little is known about Sporacetigenium, levels of this genus have been found to increase in response to Lactobacillus plantarum probiotic supplementation in humans (15), infections with Salmonella enterica and Lawsonia intracellularis in pigs (16), and high fat diets in mice (17). Sporacetigenium hydrolyzes starch and major in vitro glucose fermentation products include acetate, ethanol, H2 and CO2 (14). Sporacetigenium neither ferments starch nor produces butyrate, distinguishing this genus from most other Firmicutes genera. Elevated levels of Sporacetigenium in individuals with impaired glucose metabolism may reflect the ability of this genus to thrive in conditions where excess glucose persist.
It remains to be determined whether DRS-associated reductions in Sporacetigenium correlate with improved blood glucose and insulin levels in persons with clinical illness because the average fasting blood glucose levels for the ELD (5.6 mmol/L) and MID groups (5.4 mmol/L) fall below the diagnostic criteria for ‘prediabetes’ and Type 2 Diabetes (6.0 – 6.9 mmol/L and > 7.0 mmol/L, respectively). However, it should be noted that all individuals who eventually develop insulin resistance (leading to Type 2 Diabetes) display rising blood glucose levels. Interventions that reverse rising trends, even in healthy people, may still have positive effects by delaying or preventing levels from reaching disease conditions.
In conclusion, we report the novel finding that Sporacetigenium levels decrease as diabetic parameters improve following twelve weeks of DRS consumption in both middle-aged and elderly individuals. No other significant correlations between diabetic parameters and Firmicutes genera were detected. Finally, the correlation between high Sporacetigenium levels and high insulin levels at baseline further suggests a connection between Sporacetigenium in the gut and insulin secretion by the host. Further research exploring the relationship between prebiotics, Sporacetigenium, and blood glucose and insulin levels is warranted.
Funding information: Funding provided by MSPrebiotics Inc.
Acknowledgements: We greatly appreciate the participation of those enrolled in the clinical trial, as well as David Strang, Michael Kaan, Daryl Dyck, Jo-Ann Lapointe-McKenzie, Jean Helps, Nila McFarlane, Betty Smith (Deer Lodge, Winnipeg); Brendan Dufault, Lisa Lix (University of Manitoba); Jessica Forbes, Morag Graham, Gary Van Domselaar, Vanessa Laminman (National Microbiology Laboratory, Public Health Agency of Canada); Paramjit Tappia, Nancy Olson, Pat DeGagne, David Bray, Brenda-Lee Murray (St. Boniface Research Centre); and Brett Hiebert (Asper Clinical Research Institute).
Conflicts of Interest: Jason Bush is employed by and Michelle Alfa provides consulting services for MSPrebiotics Inc.
Ethical Statements: Research and ethics approval and protocol modification approvals were obtained from Health Canada and the University of Manitoba Research Ethics Board before implementation. Health Canada authorized the study (Submission #188517), which was listed on ClinicalTrials.gov (NCT01977183). Procedures were followed in accordance with Health Canada and University of Manitoba ethical standards. Participant identifiers were treated in confidence and according to the Personal Health Information Act of Manitoba. Study information was locked in a secured area and information sent for statistical analyses had no participant identifiers. The University of Manitoba Office, Research Quality Management unit independently audited the study.
References
1. P. D. Schloss, S. L. Westcott, T. Ryabin, J. R. Hall, M. Hartmann, E. B. Hollister, R. A. Lesniewski, B. B. Oakley, D. H. Parks, C. J. Robinson, J. W. Sahl, B. Stres, G. G. Thallinger, D. J. van Horn and C. F. Weber, “Introducing mothur: open-source, platform-independent, community-supported software for describing and comparing microbial communities,” Applied and Environmental Microbiology, vol. 75, no. 23, pp. 7537-7541, 2009.
2. S. Macfarlane and G. T. Macfarlane, “Regulation of short-chain fatty acid production,” Proceedings of the Nutrition Society, vol. 62, no. 1, pp. 67-72, 2003.
3. H. D. Holscher, “Dietary fiber and prebiotics and the gastrointestinal microflora,” Gut Microbes, vol. 8, no. 2, pp. 172-184, 2017.
4. I. Rowland, G. Gibson, A. Heinken, K. Scott, J. Swann, I. Thiele and K. Tuohy, “Gut microbiota functions: metabolism of nutrients and other food components,” European Journal of Nutrition, vol. 57, pp. 1-24, 2018.
5. H. V. Lin, A. Frassetto, E. J. Kowalik, A. R. Nawrocki, M. M. Lu, J. R. Kosinski, J. A. Hubert, D. Szeto, X. Yao, G. Forrest and D. Marsh, “Butyrate and Propionate Protect against Diet-Induced Obesity and Regulate Gut Hormones via Free Fatty Acid Receptor 3-Independent Mechanisms,” PLoS ONE, vol. 7, no. 4, p. e35240, 2016.
6. T. C. Rideout, S. V. Harding, A. Raslawsky and C. B. Rempel, “Dietary Resistant Starch Supplementation Increases High-Density Lipoprotein Particle Number in Pigs Fed a Western Diet,” Journal of Dietary Supplements, vol. 14, no. 3, pp. 334-345, 2017.
7. D. Mariat, O. Firmesse, F. Levenez, V. Guimaraes, H. Sokol, J. Dore, G. Corthier and J. Furet, “The Firmicutes/Bacteroidetes ratio of the human microbiota changes with age,” BMC Microbiology, vol. 9, p. 123, 2009.
8. M. J. Alfa, D. Strang, P. S. Tappia, M. Graham, G. Van Domselaar, J. D. Forbes, V. Laminman, N. Olson, P. DeGagne, D. Bray, B.-L. Murray, B. Dufault and L. M. Lix, “A randomized trial to determine the impact of a digestion resistant,” Clinical Nutrition, vol. 37, no. 3, pp. 797-807, 2018.
9. M. J. Alfa, D. Strang, P. S. Tappia, N. Olson, P. DeGagne, D. Bray, B.-L. Murray and B. Hiebert, “A Randomized Placebo Controlled Clinical Trial to Determine the Impact of Digestion Resistant Starch MSPrebiotic on Glucose, Insulin, and Insulin Resistance in Elderly and Mid-Age Adults,” Fronteirs in Medicine (Lausanne), vol. 4, p. 260, 2018.
10. D. W. K. N. M. Cockburn, “Polysaccharide Degradation by the Intestinal Microbiota and Its Influence on Human Health and Disease,” J Mol Biol, no. 428, pp. 3230-3252, 2016.
11. E. E. Canfora, C. M. van der Beek, G. D. A. Hermes, G. H. Goossens, J. W. E. Jocken, J. J. Holst, H. M. Van Eijk, K. Venema, H. Smidt, E. G. Zoetendal, C. H. C. Dejong, K. Lenaerts and E. E. Blaak, “Supplementation of Diet With Galacto-oligosaccharides Increases Bifidobacteria, but Not Insulin Sensitivity, in Obese Prediabetic Individuals,” Gastroenterology, vol. 153, no. 1, pp. 87-97, 2017.
12. J. J. Kozich, S. L. Westcott, N. T. Baxter, S. K. Highlander and P. D. Schloss, “Development of a dual-index sequencing strategy and curation pipeline for analyzing amplicon sequence data on the MiSeq Illumina sequencing platform,” Applied and Environmental Microbiology, vol. 79, no. 17, pp. 5112-20, 2013.
13. Y. Benjamini and Y. Hochberg, “Controlling the False Discovery Rate: A Practical and Powerful Approach to Multiple Testing,” Journal of the Royal Statistical Society. Series B (Methodological), vol. 57, no. 1, pp. 289-300, 1995.
14. S. Chen, L. Song and X. Dong, “Sporacetigenium mesophilum gen. nov., sp. nov., isolated from an anaerobic digester treating municipal solid waste and sewage,” International Journal of Systematic and Evolutionary Microbiology, vol. 56, no. Pt 4, pp. 721-5, 2006.
15. L. Y. Kwok, Z. Guo, J. Zhang, L. Wang, J. Qiao, Q. Hou, Y. Zheng and H. Zhang, “The impact of oral consumption of Lactobacillus plantarum P-8 on faecal bacteria revealed by pyrosequencing,” Beneficial Microbes, vol. 6, no. 4, pp. 405-13, 2015.
16. K. A. Borewicz, H. B. Kim, R. S. Singer, C. J. Gebhart, S. Sreevatsan, T. Johnson and R. E. Isaacson, “Changes in the Porcine Intestinal Microbiome in Response to Infection with Salmonella enterica and Lawsonia intracellularis,” PLoS ONE, vol. 10, no. 10, p. e0139106, 2015.
17. Y. R. Nobel, L. M. Cox, F. F. Kirigin, N. A. Bokulich, S. Yamanishi, I. Teitler, J. Chung, J. Sohn, C. M. Barber, D. S. Goldfarb, K. Raju, S. Abubucker, Y. Zhou, V. E. Ruiz, H. Li, M. Mitreva, A. V. Alekseyenko, G. M. Weinstock, E. Sodergren and M. J. Blaser, “Metabolic and metagenomic outcomes from early-life pulsed antibiotic treatment,” Nature Communications, vol. 6, p. 7486, 2015.